
Popular topics

-
References
Arenas E et al. (2015). How to make a midbrain dopaminergic neuron. Development. 142, 1918-1936.
Cave JW and Baker H (2009). Dopamine systems in the forebrain. Adv Exp Med Biol 651, 15–35.
Cheng HC et al. (2010). Clinical progression in Parkinson disease and the neurobiology of axons. Ann Neurol 67. 715–725.
Dorsey E R et al. (2018a). Global, regional, and national burden of Parkinson’s disease, 1990–2016: a systematic analysis for the global burden of disease study 2016. Lancet Neurol 17, 939–953.
Dorsey E R et al. (2018b). The emerging evidence of the Parkinson pandemic. J Parkinsons Dis 8, 3–8.
Gantz S C et al. (2018). The evolving understanding of dopamine neurons in the substantia nigra and ventral tegmental area. Annu Rev Physiol 80, 219–241.
Ghosh B et al. (2019). Partial reconstruction of the nigrostriatal circuit along a preformed molecular guidance pathway. Mol Ther Methods Clin Dev 14, 217–227.
Hagell P and Brundin P (2001). Cell survival and clinical outcome following intrastriatal transplantation in Parkinson disease. J Neuropath Exp Neurol 60, 741–752.
Jastrzębowska M A et al. (2019). Dopaminergic modulation of motor network compensatory mechanisms in Parkinson’s disease. Hum Brain Mapp 40, 4397–4416.
Lelos M J et al. (2016). Amelioration of non-motor dysfunctions after transplantation of human dopamine neurons in a model of Parkinson’s disease. Exp Neurol 278, 54–61.
Postuma R B et al. (2016). The new definition and diagnostic criteria of Parkinson’s disease. Lancet Neurol 15, 546–548.
Sender R et al. (2016). Revised estimates for the number of human and bacteria cells in the body. PLoS Biology 14.
Tarakad A and Jankovic J (2017). Diagnosis and management of Parkinson’s disease. Semin Neurol 37, 118–126.
Thomas B and Beal M F (2007). Parkinson’s disease. Hum Mol Genet 16, R183–R194.
Von Linstow C U et al. (2020). Does developmental variability in the number of midbrain dopamine neurons affect individual risk for sporadic Parkinson’s disease? J Parkinsons Dis 10, 405–411.
Wijeyekoon R and Barker R A (2009). Cell replacement therapy for Parkinson’s disease. Biochim Biophys Acta 1792, 688–702.
Hopes to Restore Movement in People with Parkinson’s

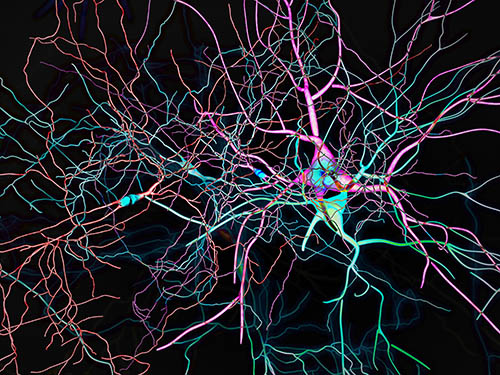
Imagine waking up from a bad night’s sleep, struggling to move, living in pain, and losing your smile. These are just some of the many symptoms experienced by people with Parkinson’s disease (PD), a neurodegenerative condition. The motor symptoms arise from the progressive death of dopaminergic neurons from the substantia nigra and subsequent depletion of dopamine in the striatum.
Emma Green is a PhD student in Neuroscience at Keele University. She is researching the possible influences of the B vitamin nicotinamide in Parkinson's disease. In this guest blog, she discusses why cell replacement therapy (CRT) may offer hope for restoring movement in people with PD.
Originally described by James Parkinson in 1817, PD is now one of the most common movement disorders, with global cases estimated at 6.1 million in 2016, increasing from 2.5 million in 1990 (Dorsey et al. 2018a), and with numbers expected to continue rising (Dorsey et al. 2018b). The pathology is well described, with dopaminergic neuron death considered to arise from abnormal α-synuclein aggregation, mitochondrial dysfunction, and oxidative stress. However the "why” remains a mystery, with the majority of cases classed as idiopathic, and only 10% having a familial link (Thomas and Beal 2007). Current treatments, typically medication, can provide some relief from symptoms (Tarakad and Jankovic 2017), but unfortunately there is no known cure or long-term treatment for PD.
Can CRT Restore Depleted Dopamine?
Perhaps the most ambitious potential treatment for the motor symptoms of PD is CRT. Much like Levodopa (the most effective medication currently used), the aim of CRT is to increase the depleted dopamine levels in the striatum. However, unlike orally taken medication, CRT would provide a constant supply of dopamine directly to the striatum by introducing dopaminergic neurons into the area de-innervated by substantia nigra dopaminergic neuron death. A number of clinical trials in the 1980s and 1990s showed the potential of CRT for PD by grafting tissue derived from the developing ventral midbrain. However, drawbacks including outcome variability, unwanted side-effects, and an unsustainable graft source, halted the progression of CRT for PD (Wijeyekoon and Barker 2009). However, these trials helped researchers to narrow down what is needed for a successful dopamine neuron graft, including survival in numbers of at least 100,000, the absence of proliferating cell types, and the expression of the genetic and phenotypic markers of substantia nigra dopaminergic neurons (Hagell and Brundin 2001). Not all dopaminergic neurons are the same, with regional heterogeneity in expression patterns throughout development and maturity both across and within forebrain and midbrain populations (Cave and Baker 2009, Gantz et al. 2018).
Now the TRANSEURO transplant study aims to assess the feasibility of CRT as a therapy for PD. Although this trial utilizes ventral mesencephalic tissue, the results will aid in the development of a standardized surgical method to deliver dopaminergic neurons, from any source, to the striatum. And there are many groups working towards providing that sustainable source of midbrain dopaminergic neurons with many suggesting embryonic and induced pluripotent stem cells as the most promising sources.
Overcoming the Challenges of CRT
Generating cells suitable for CRT in PD has been a long process with significant improvements in differentiation and reprogramming protocols made over the years. However, generating an accessible source of cells that can reliably and consistently survive transplantation into a hostile host environment, differentiate into a well characterized population primarily composed of specific dopaminergic neurons, integrate and reinnervate the striatum, without excessive outgrowth, while utilizing a short, scalable, and defined culturing process in line with good manufacturing practice guidelines, is about as easy as it sounds. Yet despite the difficulty in generating a group of cells that account for approximately 0.000002% of the human body (Sender et al. 2016, Von Linstow et al. 2020), people are making headway. We now have a more detailed understanding of their development, expression patterns, and are producing protocols with an increasing percentage (now up to 75%) of specific midbrain dopaminergic neurons (Arenas et al. 2015). And to take CRT one step further, there are even those looking to recreate the nigrostriatal pathway (Ghosh et al. 2019), which would allow grafts to be transplanted into the substantia nigra, receiving their original afferent connections while restoring the efferent connections to the striatum.
From the relatively simple idea of replacing the subset of cells lost in PD, decades of research have shown just how ambitious CRT for PD really is. Ideally, we could protect these dopaminergic neurons. However, there are no objective tests that can identify PD without the presence of motor symptoms (Postuma et al. 2016), which occur after degeneration of a third of the dopaminergic neurons in the substantia nigra (Cheng et al. 2010). This dissociation between initial dopaminergic neuron degeneration and later motor symptom presentation is thought to occur due to compensatory mechanisms in the basal ganglia (Jastrzębowska et al. 2019), masking the onset of dopaminergic neuron degeneration. This, alongside a prodromal phase with symptoms such as constipation, sleepiness, and depression, which are shared with many other diseases, means that diagnosis of PD often occurs at a point when substantial degeneration has already occurred and neuroprotective therapies may be ineffective. Therefore, until the identification of both pre-symptomatic tests and effective neuroprotective therapies, CRT holds the potential to reverse some of the motor symptoms experienced by people with Parkinson’s and restore their freedom of movement. An in vivo PD model has shown hope that CRT could also alleviate some of the non-motor features of striatal dopaminergic denervation (Lelos et al. 2016). Improving the motor symptoms experienced by people with Parkinson’s may also contribute to improvements in some non-motor symptoms such as fatigue and mood. Furthermore, CRT could also provide relief from medication and the detrimental side effects associated with their intake. While we are still some way off being able to offer CRT to patients, this potential development gives hope for the future and we eagerly await the results of the TRANSEURO transplant study.
Researching Neurodegenerative Diseases?
At Bio-Rad, we have made neurodegenerative diseases a key focus area, with reagents that include a panel of antibodies to the Huntingtin protein, the highest cited clones for research into protein gene product (PGP) 9.5, and antibodies to amyloid beta 1-42 for staining amyloid plaques in Alzheimer’s Disease brains.
View Our RangeReferences
Arenas E et al. (2015). How to make a midbrain dopaminergic neuron. Development. 142, 1918-1936.
Cave JW and Baker H (2009). Dopamine systems in the forebrain. Adv Exp Med Biol 651, 15–35.
Cheng HC et al. (2010). Clinical progression in Parkinson disease and the neurobiology of axons. Ann Neurol 67. 715–725.
Dorsey E R et al. (2018a). Global, regional, and national burden of Parkinson’s disease, 1990–2016: a systematic analysis for the global burden of disease study 2016. Lancet Neurol 17, 939–953.
Dorsey E R et al. (2018b). The emerging evidence of the Parkinson pandemic. J Parkinsons Dis 8, 3–8.
Gantz S C et al. (2018). The evolving understanding of dopamine neurons in the substantia nigra and ventral tegmental area. Annu Rev Physiol 80, 219–241.
Ghosh B et al. (2019). Partial reconstruction of the nigrostriatal circuit along a preformed molecular guidance pathway. Mol Ther Methods Clin Dev 14, 217–227.
Hagell P and Brundin P (2001). Cell survival and clinical outcome following intrastriatal transplantation in Parkinson disease. J Neuropath Exp Neurol 60, 741–752.
Jastrzębowska M A et al. (2019). Dopaminergic modulation of motor network compensatory mechanisms in Parkinson’s disease. Hum Brain Mapp 40, 4397–4416.
Lelos M J et al. (2016). Amelioration of non-motor dysfunctions after transplantation of human dopamine neurons in a model of Parkinson’s disease. Exp Neurol 278, 54–61.
Postuma R B et al. (2016). The new definition and diagnostic criteria of Parkinson’s disease. Lancet Neurol 15, 546–548.
Sender R et al. (2016). Revised estimates for the number of human and bacteria cells in the body. PLoS Biology 14.
Tarakad A and Jankovic J (2017). Diagnosis and management of Parkinson’s disease. Semin Neurol 37, 118–126.
Thomas B and Beal M F (2007). Parkinson’s disease. Hum Mol Genet 16, R183–R194.
Von Linstow C U et al. (2020). Does developmental variability in the number of midbrain dopamine neurons affect individual risk for sporadic Parkinson’s disease? J Parkinsons Dis 10, 405–411.
Wijeyekoon R and Barker R A (2009). Cell replacement therapy for Parkinson’s disease. Biochim Biophys Acta 1792, 688–702.
You may also be interested in...

View more Neuroscience or Guest Blog blogs
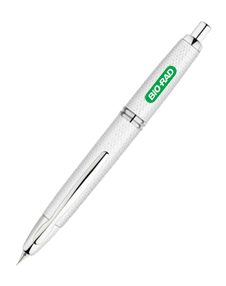
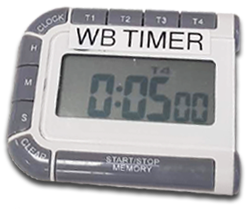
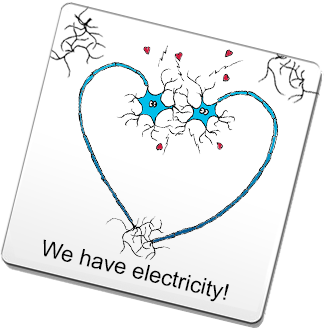